Introduction
Composites are materials made of at least two different materials. In structural applications, fibre-reinforced plastics - in which the fibres have been dispersed into a matrix - are commonly used.
The term "composite" not only includes fibre reinforced plastics but a whole series of materials or products composed of different components (concrete, asphalt, ceramic composites...).
Fibre-reinforced polymer materials consist of fibres, which have high strength and modulus, bonded to a matrix, which may have different physical and chemical identities.
Properties
FRP possess excellent mechanical properties:
- Impact resistance
- Strength
- Stiffness
- Flexibility
- Ability to carry loads
Typical tests used to measure the mechanical properties of FRP:
- Shear stiffness
- Tensile strength
- Flexible Modulus
- Impact resistance
The mechanical properties of FRP are determined by different factors including:
- the composition of the matrix: chemical composition, crystallinity, curing, presence of additives, etc.
- Fibre composition (glass, carbon, aramid) and fineness
- textile structure: individual fibres, continuous yarns (rovings), fibre-based textiles including hybrid yarns, fibre mats, spunlaid yarn layers (non crimp fabrics) 3D textiles (knits, braids...)
- Fibre concentration
- Fibre length
- Adhesion between matrix (resin) and fibre
- Fibre orientation
- Heat and humidity
Influence of high strength fibres on FRP properties
Adding high strength fibres to the plastic (resin) matrix not only changes the strength of the FRP but also other properties:
Mechanical behaviour
Reduction of crimp and warping behaviour at high temperatures and of creep under (continuous) load.
Burning behaviour
Certain high strength fibres such as glass and basalt fibres are highly resistant to fire and are therefore suited to improve the burning behaviour of plastics.
Thermal and electric conductivity
Thermally and electrically conductive fibres (eg. carbon fibres) will affect the inherently good insulating properties of most plastics.
Carbon fibres in panels or structures may alter the detectability by radar waves (the radar transparency of CRP is used in military airplanes) and can also form a Faraday Cage to protect sensible electronic devices.
The presence of heat conductive fibres (eg. CF) can also accelerate the thermal process of forming and curing polymer systems.
- the thermal conductivity of the fibres can improve the heat transmission in conventional ovens to and within the composite structure
- carbon fibres can also capture electric waves allowing the application of inductive heating systems that will decrease the processing time.
Thermal insulation
Most plastics and high strength fibres do not conduct heat (or conduct it only very poorly). This thermal insulation of FRPs can be marketed as an advantage in contrast to materials reinforced with metal rods or cables. Many heat losses are indeed due to the presence of thermal bridges.
Corrosion resistance
In contrast to most metal reinforcing materials (metal reinforced concrete), most reinforcing fibres and polymer matrices are corrosion-free, which makes them very suited for several building applications (pipes, reservoirs...).
Colour and resistance to ageing
Fibres can of course influence the colour of the composite (carbon fibres are black and basalt fibres have a golden hue) as well as the resistance to ageing.
Affinity to functionalisation
Fibre reinforced plastics can be easily functionalised by means of coating, printing, laminating which may be advantageous in certain applications. It is possible to integrate smart materials and sensors.
RFID and other communication systems can be integrated by means of IML (In-Mould Labelling) techniques increasing the applicability of FRPs in new domains.
In a digitalised world governed by the "Internet of Things", functionalised composites will be playing a major role.
Sustainable FRP application domains
Transportation
The transportation of people and goods depends on several means and products including:
- infrastructure: roads, bicycle paths, airports, railroads, sound barriers...
- transportation means: cars, bicycles, buses, trains, airplanes, funiculars...
- communication means: signalisation panels, traffic lights, GPS (satellites)
- other auxiliary goods: containers, packaging...
In most of these market segments, FPRs can be applied. Thanks to their specific properties such as light weight they may reduce the environmental impact linked to mobility and transportation. By reducing the weight of transportation means one can significantly restrict fuel consumption and hence the CO2 emission of cars, trucks and planes.
Major aircraft manufacturers have already greatly reduced the weight of their airplanes by substituting metal components by carbon fibre reinforced plastics and other composites in structural and non-structural components.
Although the car industry has also replaced non-structural metal components by FRPs, the overall weight of cars has not been reduced. More powerful engines and the incorporation of comfort and safety systems have even added to the final weight of cars. However, by using FRPs this increase remains within boundaries.
The great challenge is not limited to the application of structural composites in cars, but also involves the development of "smart" composites able to take over a whole series of electrical, mechanical and electronical functions that are now mainly provided by heavy and complexly composed systems.
Renewable energy systems
Available and affordable energy is essential to ensure our comfortable way of life. House heating systems, daily household activities, mobility, industrial production, medical examinations... all depend on massive energy consumption. Because this modern and energy intensive society is shared with more and more people on this planet, energy supply is a major societal challenge.
At this very moment, most of our energy supply is still based on the exploitation of exhaustible energy resources (fossil fuels, gas, coal). To secure the energy supply in the long run as well as to reduce the environmental impact and health risks linked to it, a massive switch to renewable energy sources and low energy processes is required.

Wind energy
The first windmills of the 1980's had a rotor diameter of about 15m and a relatively small power output (50 to 100kW). In 2013, the average windmill rotor had a diameter of about 100m generating about 2,5MW. A two megawatt wind turbine generates an average of 4 million kWh/year, which corresponds with the energy consumption of 1300 households.
The increasing size of wind turbines has several consequences:
- Only a small number of companies are capable of producing these large (mainly offshore) wind turbines. Today, the European offshore wind energy market is dominated by Vestas Wind Systems and Siemens Wind Power (together they have realised 90% of the installed wind power). Other European companies are REpower, Bard and Areva.
- Due to the growing size, the glass fibre reinforcement has to be supplemented by carbon and aramid fibre reinforcement and with more performant core materials.
- The production techniques (in particular the infusion and prepreg technologies) and infrastructure need to be adapted to produce the very long blades (with a length of more than 60 m) economically and ecologically. One of the main challenges is avoiding the formation of voids in large surfaces and the homogeneous and quick curing of thermoset resins.
- Finally, the transportation and mounting of these very long blades require adapted procedures and working methods.
The use of appropriate FRP and core materials adapted to the task are essential in further developments of rotors and blades.
Desirable material improvements:
- Protection of the blade structure and its mechanical properties against lighting strikes by the integration of electric conductors in the blades. Damage by lightning is a major cause of standstill and loss of output. Some installations are equipped with monitoring systems to keep track of the number of lightning strikes.
- Integration of measuring systems in the composite material to enable online monitoring of tensions and impact. In principle, the minimal life span of windmills is twenty years, during which the desired output and a minimum of maintenance costs are to be expected.
- Detection systems to rapidly detect damage to the components and the possible integration of self-healing systems to automatically repair damages.
- Invisibility to radar waves. The communication of civil and military aircrafts can be disturbed by wind farms. To do this in a economical responsible way is a real challenge.
Solar energy systems
- Solar cells: PV (Photovoltaic) and PVT (Photovoltaic Thermal) panels convert solar energy (radiation) in electricity. In contrast to PV, a PVT panel also generates heat.
- Thermo-solar steam turbines: heat is formed by the concentration of solar light and transmitted to a fluid (thermal oil or water) to generate steam. This steam produces electricity. These systems are mainly used in southern Europe and Africa.
- Solar water heating (SWH): the harvested sun beams are used to heat water. By means of a heat exchanger and a pump the water is circulated.
In most solar energy systems, FRPs can be used. PV and PVT panels and SWH systems can be incorporated in lightweight FRP profiles reducing the load on the roof. It is also possible to use foam components (core materials also used to enhance the rigidity of FRPs) in the supporting composite structures to enhance the efficiency of the solar energy system.
Corrosion-free GRP composites are very suited for SWH: pipes and reservoirs made from composite materials are indeed highly resistant to (very) hot and mostly corrosive water.
Maritime energy
Seas and oceans enclose energy that can be harvested by
- Tidal power stations: Tidal forces are periodic variations in gravitational attraction exerted by celestial bodies. These forces create corresponding motions or currents in the world's oceans. Due to the strong attraction to the oceans, a bulge in the water level is created, causing a temporary increase in sea level.
- Wave power stations capture the energy generated by wind passing over the surface of the sea. As long as the waves propagate slower than the wind speed just above the waves, there is an energy transfer from the wind to the waves. Both air pressure differences between the upwind and the lee side of a wave crest, as well as friction on the water surface by the wind, making the water to go into the shear stress causes the growth of the waves.
- Ocean thermal energy conversion (OTEC) uses the temperature difference between cooler deep and warmer shallow or surface seawaters to run a heat engine and produce useful work, usually in the form of electricity.
The enormous forces at sea and the influence of seawater can damage the floating (offshore) systems. Fibre reinforced plastics are stronger and more resistant to corrosion than steel are therefore very suited materials for these applications (stations, structures and piping...).
Sustainable construction with FRPs
The construction market not only includes the construction of private or public buildings, but also other infrastructural constructions such as viaducts, roads, bridges, car parks, harbours, pipelines, etc. Although it is an important consumer of FRPs, the sales volume of glass-fibre, carbon-fibre reinforced plastics (GRP and CRP) as well as of Wood-Plastic Composites (WPC) still remains rather low in comparison with other construction materials.
This is mainly due to a lack of a profound knowledge of the advantages of FRPs, especially in the field of sustainability:
- Reduced CO2 emission during the transportation of lightweight composites
- Deployment of less heavy equipment (cranes, trucks, forklifts…) during construction
- Thermal insulation
- Freedom of design
- Affinity to functionalisation
- The resistance to corrosion and rot of FPRs and (consequent) energy savings that can be realised (production, transportation, installation, maintenance, longevity) are major advantages of FRPs in comparison with conventional materials (reinforced concrete, steel, wood…).
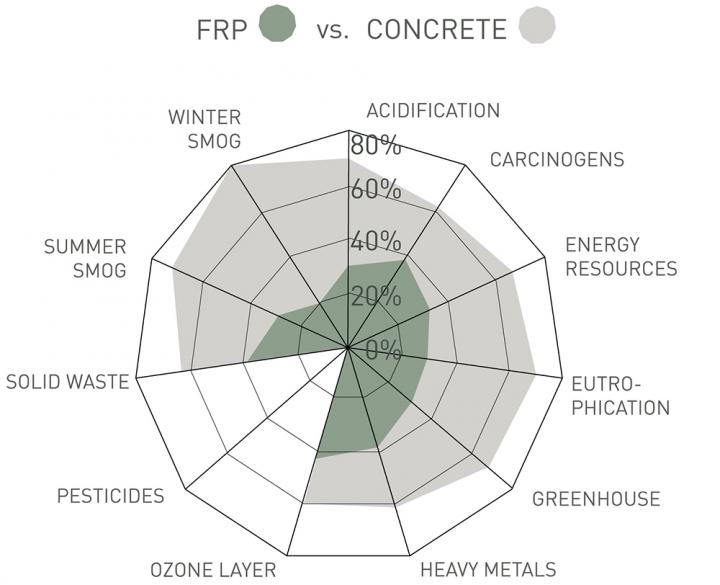
Sustainability is a popular and important issue facing designers, builders and building owners. Unfortunately, it is also complex. Recent developments have shown that programs such as LEEDS assessments do not always reveal the correct or best decisions about material selection or building strategies. The more appropriate but also more rigorous method is “Life Cycle Assessment” or LCA’s where a material’s total, cradle to grave environmental impact is compared to an alternative. These exercises are complicated and project specific. For example, often the weight of a product plays a significant role in impacts like transportation, installation, and back-up structure. All these factors vary from project to project and to measure all these factors is usually beyond the scope of most conventional construction projects.
Source: Kreysler & Associates
Innovation opportunities for FRPs for the construction market:
- Parking space floor with incorporated wireless inductive charging system for electric vehicles: A user-friendly method to charge a car's batteries is based on inductive energy transfer. The floor on top of the inductive charging system may not block the energy tranfer and may therefore contain no conductors. Carbon (and also glass) fibre reinforced concrete floors or road covering zones have already been proven very successful.
- Inductive energy transfer also allows the charging of battery-free automated guided vehicle (AGV) systems, making battery charging stations redundant in companies. The energy necessary to actuate these transportation systems is being supplied by inductive energy transfer systems that are embedded in the FRP floor covering.
- EMI-shielding by the integration of very thin electrically conductive FRP panels (based on carbon fibres) into building elements (walls, ceilings and floors).
- Energy storage materials by combining both conductive and insulating FRPs. Volvo already applies this principle to use the car body as a battery. The same system could be used to store energy generated by solar panels in FRP mural or ceiling panels.
- Co-extruded mural or ceiling profiles based on electrically conductive carbon fibre reinforced plastics (at the inside) and electrically insulating plastics (at the outside). This system allows to insert and connect several electric systems (LED, alarm systems...) in a single profile.
- Green building
- CF FRP-wrapping technology to repair and improve existing infrastructure (bridges, viaducts, railroads...) at lower costs
- Retrofitting with improved resistance to fire
- Integration of smart materials
- PCM: energy storage and release based on phase change materials
- conductive polymers (eg. in solar panels)
- self-cleaning, self de-icing and self-healing FRP cladding
- FRP coatings with sensor functions
FRPs in healthcare
The strength, lightweight and adaptable/flexible design of FRPs are major assets in the development and production of "assistive auxiliary devices". The growth of this market segment is linked with the ageing of the population. To keep the healthcare related costs under control it is important that the elderly live at home as long as they possibly can. This requires, however, that several appropriate devices are available to them that will assist them to answer their mobility, communication and personal healthcare needs.
By using carbon fibre reinforced plastics - instead of metal components - it is possible to reduce the weight of both simple (spectacle frames, walking canes...) and complex devices (wheelchairs, rollators, walking assistant robots...). Reducing their weight while preserving their necessary strength will simplify their use, folding, storing...
The design of assistive devices, especially the ones used during care activities, must be adaptable to answer to a person's comfort related and ergonomic needs.
The affordability of devices within the healthcare sector remains a major challenge.
Recycling and valorisation
FRPs, in general, are difficult to recycle due to their multiphase nature, typically containing three or more components: fibre reinforcement, resin matrix and fillers (typically calcium carbonate, CaCO3). Various recycling technologies have been proposed but none have proved economically viable. In many cases, the price of raw material produced from recycled composites is considerably higher than the prices for virgin reinforcements and fillers.
Recycling of thermoset FRPs presents an especially difficult challenge because once the thermoset matrix molecules are cross-linked, they cannot be melted or reformed. Thermoplastics, on the other hand, are inherently recyclable. Further, the value of the material constituents of GRP reclaimed from recycling is low. Hence there is little monetary incentive to recycle.
FRP waste streams include both "Post Production" (from production, assembling) and "Post Consumer" waste or EOL (discarded, defective products and materials).
Because of the long lifespan of FRP products (expected lifespan of wind turbines is estimated at ± 25 years, buildings > 50 years, aircrafts > 30 years) with the usual exceptions to the rule (cars ± 10 years, sporting articles ± 5 years), E+E (< 5 years) - there are no large quantities of FRP-EOL wastes available for recycling and valorisation. The selective collection is not obvious since many FRPs are components of larger items (cars, devices, installations, constructions...). In addition, many FRPs are hardly to distinguish visually from regular plastics.
Recycling companies are not (yet) equipped to identify, dismantle and sort FRPs products for further recycling (eg. robotisation for dismantling C-waste).
European legislation is steering the recycling of both PP and PC-FRP waste streams, focusing on:

FRP Recycling and valorisation routes
Challenges and opportunities
Recycling of regular FRPs with the existing recycling techniques is only economically viable in the case of sufficiently large quantities.
The story of carbon fibre (CF) reinforced plastics is a totally different one. Presently available technologies allow the production of valuable recycled carbon fibres (r-CF) extracted from carbon fibre reinforced plastics (CFRPs) of a quality answering to the expectations of the suppliers to the automotive industry regarding their application in the production of fibre-reinforced thermoplasts and thermosets.
Compatibilizers
Compatibilizer additives allow resins that would not neatly blend and bond in a way that creates enhanced performance when compared with either polymer alone. The use of compatibilizers is being explored increasingly in the recycling industry as a way to create value in mixed feed streams.
Compatibilizers have a hybrid nature: part of it is compatible with substance A, another part with substance B. Compatibilization is based on the physico-chemical interactions of these substances with the hybrid material.